Transformative nanomaterial synthesis using biological conditions
使用nanomat时承诺和关注的一个领域erials is their potentially high level of reactivity. This comes from the massively increased surface area of the material when it is segmented into much smaller pieces. A copper penny in your hand, or in a biological liquid, is much less reactive than that same mass of copper chopped into many, many, small nanoparticles. Furthermore, a larger metallic surface (such as the penny) has had time to age and to be less reactive than the copper nanoparticles, which may have aggregated and not been “revealed” until interacting with a cell. Finally, nanoparticles are of a small enough size that they more efficiently interact with cells compared to much larger objects, by actually penetrating them or by being ingested by the cells. This higher reactivity of nanoparticles provides us with tools to target cells for destruction, such as in cancer, and for promoting cell growth, as in tissue engineering and regeneration in healing.
Because of the potential for cell killing using metallic nanoparticles such as copper, we added different concentrations of these copper nanoparticles (CuNPs) to brain tumor cells in cell culture experiments. We found that increasing concentrations of CuNPs killed the tumor cells. However, unexpectedly, we discovered that when slightly less CuNPs were used, and not all of the cells were killed, large, micro-scale linear structures were formed after 2-4 days in culture. These structures were dense and of a dark color, consistent with the starting CuNPs, but were much bigger, and of a much different shape, like the spines of sea urchins (See Figure 1). Furthermore, as these larger “urchins” developed, the smaller CuNPs disappeared.
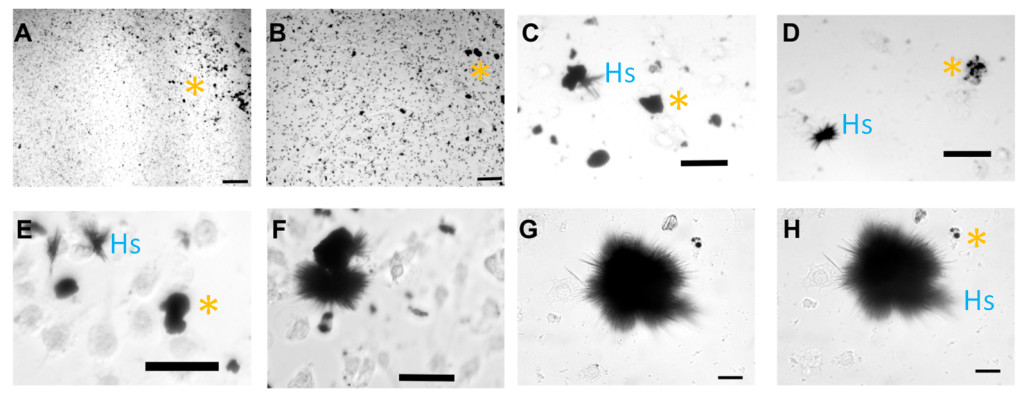
图1。转换的铜纳米颗粒(坎昆Ps) to high-aspect ratio structures (HARs) under biological conditions. CuNPs were interacted with brain tumor cells continuously as shown from time 0 (A+B), and then 17, 24, 36, 49, 69, and 82 hours under biological conditions (body temperature and physiological pH) in panels C-H, respectively. CuNPs (A&B) transformed over time into aggregates (C&D), which increasingly show linear spines (E&F), much like a sea urchin (G&H). The “*” symbols indicates clumped CuNPs, and “Hs” indicates example HARS. Scale bars are indicated in the bottom right of each figure and =100 microns for A+B, 50 microns for C-E, and 25 microns for F+G. (Adapted from the original publication by Cotton Kelly et al. in JOVE 2015).
We hypothesized that something in the cell culture and the cell culture conditions was promoting the transformation of CuNPs into more linear, high-aspect ratio structures (HARS). To test this, we subtracted substances from the cell cultures, and discovered that the key component was the amino acid cystine, which is a dimer of two cysteine molecules. The two single sulfurs in cysteine come together to form a disulfide bond in the cystine, and this appears to be essential in binding the copper from the CuNPs to form the HARS, because sulfur-containing compounds have been reported to bind copper previously, but never under these biological conditions. Another remarkable discovery during our synthesis, was that the HARS formed with simply CuNPs and cystine (and no other components), under biological conditions, formed HARS that did not clump together, and had almost identical shapes every time (See Figure 2). What did vary was the length of the HARS, which ranged from hundreds of nanometers to many microns, with diameters often in the nanometer range.
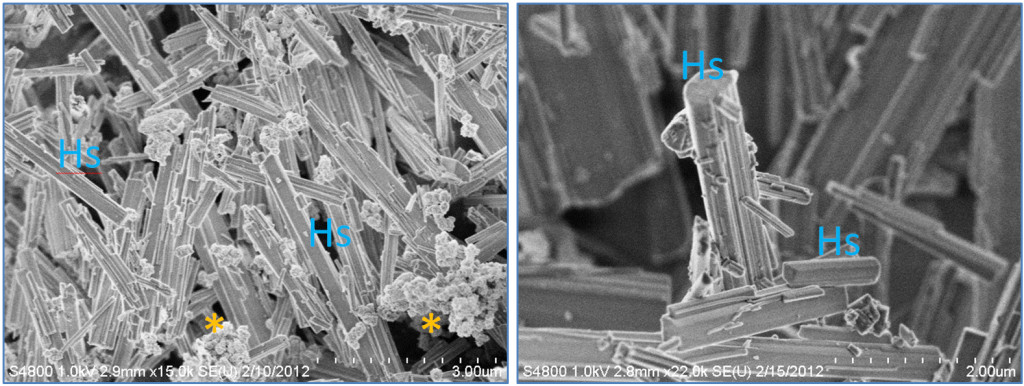
Fig.2. Nano- and Micro-scale features of discovered new synthesis for high-aspect ratio structure (HARS) biocomposites. Both panels show scanning electron microscopy images. Left panel= HARS generated from copper nanoparticles (CuNPs) and right panel= HARS generated from Copper sulfate. Scale bars in bottom right of each figure are 3 and 2 microns, with each gradation= 300 and 200 nanometers for left and right panels, respectively. The “*” symbol indicates clumped CuNPs, and “Hs” indicates example HARS. (Modified from the original publication by Cotton Kelly et al. in JOVE 2015).
Once we discovered a new transformation of CuNPs into long HARS with both micrometer and nanometer dimensions, we wondered if more fully dissociable copper sources, such as a copper salt, could also work. We hypothesized that the resulting HARS synthesis would be cleaner, since copper salts could be fully dissolved in liquid and then combined with cystine as we had done with the CuNPs. Indeed we did make this discovery, using the copper salt from copper sulfate, combined with cystine. The HARS formed from this combination were much cleaner than those from CuNPs, meaning there were no particles left over since the salt goes fully into solution (See Figure 2, right panel). Since these new reactions that we have discovered can be carried out using biologically friendly conditions, meaning at body temperature and in pH around 7.4, we hypothesize that these new metallic-composites could be used for better drug delivery and tissue engineering.
Publication
Generation of Scalable, Metallic High-Aspect Ratio Nanocomposites in a Biological Liquid Medium.
Cotton Kelly K, Wasserman JR, Deodhar S, Huckaby J, DeCoster MA
J Vis Exp. 2015 Jul 8
Leave a Reply
You must belogged into post a comment.